Thermal Energy Storage
New CommercialWhat is Thermal Energy Storage?
Thermal energy storage (TES) stockpiles thermal energy by heating or cooling a storage medium for later use in heating and cooling applications and power generation.[1] Combined Heat and Power (CHP) systems can utilize thermal energy storage to manage energy loads and reduce peak demand or to provide backup power (see Energy Storage and Backup Power, Combined Heat and Power (CHP), Demand Response and Peak Load Management). In the building sector, TES systems supply thermal energy for domestic hot water, space heating, and air-conditioning. The solar industry utilizes TES to store energy for concentrating solar power (CSP) plants, which use mirrors to focus sunlight and produce and store high-temperature thermal energy for electricity production in the absence of sunlight.[2]
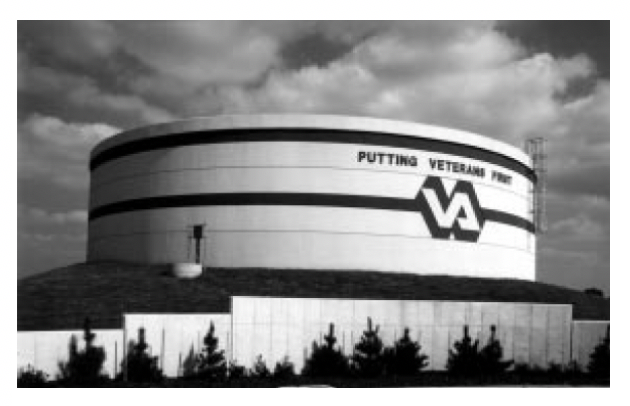
Figure 1 – Stratified water thermal storage system at Veterans Affairs Medical Center (Source: US DOE).
There are three kinds of TES systems:
- Sensible heat storage, which stores thermal energy by heating or cooling a liquid or solid storage medium (e.g., water, sand, molten salts, rocks).
- Latent heat storage, which uses phase change materials or PCMs (e.g., from a solid state into a liquid state).
- Thermo-chemical storage (TCS), which uses chemical reactions to store and release thermal energy.[3]
Sensible heat storage and latent heat storage are the most widely used for commercial building applications. Currently more complicated and cost prohibitive, future advances in thermochemical heat storage (THS) systems hold the potential for high storage density, low volume requirements, low heat loss, longer storage periods and low charging temperature.[4]
TES technologies for heating applications include electrical or solar thermal water heating systems that use hot water tanks to store water until it is needed. TES systems improve the energy efficiency of buildings and provide cost savings by storing waste heat or by-product heat when it is available and supplying it upon demand.[5] Typical independent parameters that characterize TES systems in use are shown in the below table.[6]
TES technologies for cool storage include:
- Sensible heat storage systems, such as chilled water (CHW) TES, and low-temperature fluid (LTF) TES, which store thermal energy as a temperature change.
- Latent heat storage systems, such as ice TES, which store thermal energy as a change of phase material (e.g., water to ice).
How to Integrate a Thermal Energy Storage System
First, assess whether the building meets one or more of the following conditions for integrating TES: high utility demand costs, high peak-use utility costs, high daily load variations, short durations of loads, infrequent cyclical loads, and available incentives or rebates.[7]
Next, determine what type of TES system to install based on the building size and utility costs. Phase change TES system typically work for small buildings while larger buildings often utilize temperature change systems. For example, small commercial buildings with space constraints may want to consider ice storage systems designed with integrated roof-top mounted, direct-expansion cooling systems. For larger commercial buildings, think about a stand-alone water chiller system with high thermal storage capacity. TES technology also scales well to district energy systems that serve multiple buildings.
Final steps include installation and monitoring performance to fine-tune the system and to calculate payback periods and cost-savings.
Examples
NREL’s Energy Systems Integration Facility (ESIF), Golden, CO.
The Energy Systems Integration Facility (ESIF) at the US DOE’s National Renewable Energy Laboratory (NREL) houses a state-of-the-art high-performance computing data center built to LEED Platinum. Hot water loops harvest waste heat from the data center and provide heating for the offices and labs.[8] Data center waste heat also heats glycol loops located under the plaza and walkway, melting snow and improving walkway safety.[9] Utility rebates supported building commissioning, performance verification, and system improvements.
TIAA-CREF, New York City, NY
The Teachers Insurance and Annuity Association (TIAA) replaced an aging chiller system with a new rooftop, chilled water system for ice production that runs during off-peak hours and includes a 30-tank thermal storage system.
Durst Organization, New York City, NY
The 41-story Durst Organization headquarters building uses off-peak, nigh-time electricity to make and store ice in 28 storage tanks located in the basement. Small pumps move ice-cooled fluid past fans that blow cold air into the building during daytime operations.
Benefits
Thermal Energy Storage (TES) systems facilitate participation in demand response programs, reducing peak demand, energy consumption, CO2 emissions, and costs by utilizing less expensive, off-peak electricity and by increasing the overall efficiency of energy systems (see Demand Response and Peak Load Management). TES systems improve grid reliability by shifting peak heating and cooling loads from periods of high-demand to low-demand, eliminating the need for building new or maintaining older, less-efficient standby energy plants, and providing backup power to the grid like a generator and supporting distributed renewables generation.
Costs
TES saves money on electricity and maintenance costs depending on the design of the system, the cooling, and heating requirements of the space, and the time of day during which the storage unit uses energy for its operation. Ideally, TES systems remove thermal energy from the medium during off-peak periods and circulate it during periods of high heating and cooling demand.[10]
TES systems increase in effectiveness when existing electricity demands vary during the day, as the heat capacity of the medium enables the spreading out of electricity demands more than would be possible with a traditional cooling system. As a result, TES systems work most effectively in buildings experiencing peaks in electricity demands throughout the day and in a location with high peak rates for electricity.[11]
Prices vary depending on the type of TES system selected, variations in site-specific conditions and individual vendor offerings, but annual energy savings can offset the system costs within ten years.[12] Also, financing and incentives are sometimes available from utility companies to implement sizeable thermal energy storage systems. For example, the New York State Energy Research and Development Authority (NYSERDA) helped fund the TIAA-CREF building mentioned above.
While the cost-savings of shifting load to off-peak hours drives adoption of most TES systems, designs that reduce chiller size and cost and improve building system efficiency make TES systems for customers with a flat electricity rate a viable option as well.[13]
Check with the New Jersey Clean Energy Program’s Combined Heat & Power Program, Pay for Performance Program, and NJ SmartStart Buildings Programs for available incentives and rebates.
Resiliency
TES systems offer a redundant building system for sustaining uninterrupted power in the event of a power outage. In the case of an emergency, TES systems provide a backup resource to supply heating or cooling to critical areas of a building. TES systems can complement traditional backup generation as part of an islandable microgrid and provide backup power during a grid outage or mechanical failure.[14] By contributing to the downsizing of chillers and HVAC equipment, TES systems reduce the stress on the grid during severe winter weather and heat waves, contributing to grid reliability. By shifting energy loads to off-peak hours, TES systems encourage the use of clean and renewable energy sources.
[1] International Energy Agency Energy Technology Systems Analysis Programme (IEA-ETSAP) and International Renewable Energy Agency (IREA). January 2013. Thermal Energy Storage Technical Brief. https://www.irena.org/DocumentDownloads/Publications/IRENA-ETSAP%20Tech%20Brief%20E17%20Thermal%20Energy%20Storage.pdf (accessed April 11, 2018).
[2] Mehos, Mark, et al. 2016. On the Path to SunShot: Advancing Concentrating Solar Power Technology, Performance, and Dispatchability. Golden, CO: National Renewable Energy Laboratory. http://www.nrel.gov/docs/fy16osti/65688.pdf (accessed April 13, 2018).
[3] IEA-ETSAP and IREA. Thermal Energy Storage Technical Brief. https://www.irena.org/DocumentDownloads/Publications/IRENA-ETSAP%20Tech%20Brief%20E17%20Thermal%20Energy%20Storage.pdf (accessed April 11, 2018).
[4] Devrim Aydin et al. The latest advancements in thermochemical heat storage systems. Renewable and Sustainable Energy Reviews. Vol 41. Jan 2015. Pages 356-357. https://www.sciencedirect.com/science/article/pii/S1364032114007308?via%3Dihub (accessed April 13, 2018).
[5] IEA-ETSAP and IREA. Thermal Energy Storage Technical Brief. (accessed April 11, 2018).
[6] Sarbu, I.; Sebarchievici, C. A Comprehensive Review of Thermal Energy Storage. Sustainability 2018, 10, 191.
[7] IEA-ETSAP and IREA. Thermal Energy Storage Technical Brief.
[8] IEA-ETSAP and IREA. Thermal Energy Storage Technical Brief.
ntegration Facility (ESIF).” High-Performance Buildings. Spring 2015. http://www.hpbmagazine.org/attachments/article/12185/15S-Energy-Systems-Integration-Facility-Golden-CO.pdf (accessed April 12, 2018).
[9] Michael Sheppy et al. “Energy Integration – Energy Systems Integration Facility (ESIF).” High-Performance Buildings. Spring 2015.
[10] NJ Department of Environmental Protection. “Infrastructure Energy Efficiency” http://www.state.nj.us/dep/opsc/docs/Infrastructure_Energy.pdf (accessed April 11, 2018).
[11] US DOE. Federal Energy Management Program. “Thermal Energy Storage for Space Cooling.” https://www.osti.gov/servlets/purl/770996 (accessed April 13, 2018).
[12] US DOE. Federal Energy Management Program. “Thermal Energy Storage for Space Cooling.” https://www.osti.gov/servlets/purl/770996 (accessed April 13, 2018).
[13] Ibid.
[14] NREL. Analysis Identifies Where Commercial Customers Might Benefit from Battery Energy Storage. https://www.nrel.gov/news/press/2017/where-commercial-customers-benefit-from-battery-energy-storage.html (accessed April 13, 2018).
Resources
- Energy Conservation through Energy Storage
- National Renewable Energy Laboratory (NREL) Battery and Thermal Energy Storage
- New Jersey’s Clean Energy Program – Commercial Buildings
Pacific Northwest National Laboratory
- Energy Efficiency and Renewable Energy Program
- The Role of Energy Storage in Commercial Buildings – Preliminary Report
US Department of Energy