On-Site Renewable Energy Systems
Existing CommercialWhat are On-Site Renewable Energy Systems?
Renewable energy refers to energy produced from a fuel that regenerates or does not deplete natural resources. On-site renewable energy systems for existing commercial buildings include solar, wind, geothermal, and biomass. Electricity, heating, cooling, and other applications, including multi-purpose components can utilize renewable technologies. For example, installing solar photovoltaic (PV) panels on parking structures provides shading to cars and comfort to their occupants, while providing clean electricity.
Many locations – building and/or site — can use on-site renewable energy systems. The practicality of most renewable energy systems or combination of systems for a specific construction project increases when considered early in the planning stages of the site and building design, although retrofits also may be advantageous as illustrated in Figure 1.
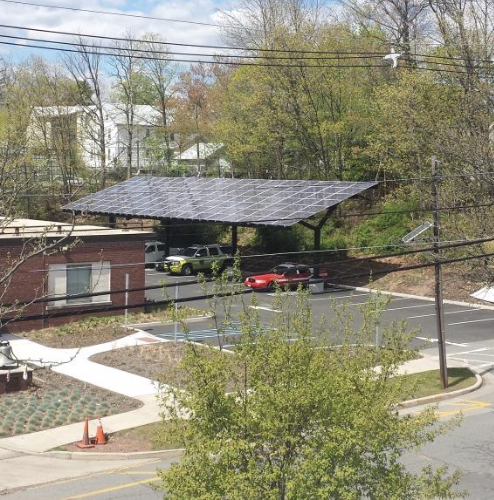
Figure 1 – Fire Station Solar Array, Highland Park, NJ. (Source: Rutgers Center for Green) Building).
Implementation of Renewable Energy Systems
Renewable energy systems are complex and require project team members with on-site renewable energy system expertise. The US DOE’s Federal Energy Management Program’s Guide to Integrating Renewable Energy in Federal Facilities offers advice on understanding renewable energy options, selecting appropriate types of renewable energy technologies, and integrating these technologies into all project phases. For implementation in New Jersey, project teams should contact the NJ Office of Clean Energy to learn about current programs, tools, and available funding. Critical project-specific variables to consider include location, space, energy costs, available project incentives, local net metering and interconnection policies. Pre-implementation steps for an on-site renewable energy system project comprise preliminary screening, a renewable energy feasibility study, sizing and design of systems. [1]
On-Site Renewable Energy Systems and Geothermal Systems
Photovoltaics (PV)
Photovoltaic (PV) systems refer to the use of renewable solar energy to create electricity. PV cells placed together in a module are grouped together electrically to form an array. Arrays of PVs applied to surfaces, such as a roof, capture light energy from the sun and produce electricity. The flexibility of installing this on-site renewable energy system makes it a suitable option for existing buildings. A specific PV system known as Building-Integrated Photovoltaic (BIPV)[2] uses PV technology and applies it to building materials, serving multifunctional purposes. For example, a BIPV skylight not only captures energy, generating electricity for a building, but also provides daylighting (see Daylighting). Another strategy incorporates PV technology into an exterior wall for decorative purposes as well as collecting energy.
New Jersey has one of the fastest growing markets in PV technology.[3] The availability of PV combined with available incentives facilitates the design and installation of PV systems in New Jersey. While projects can tailor PV systems to meet the needs of a particular site and building, south facing surfaces not shaded by nearby trees, buildings, and structures provide the best results. If possible, orient collectors at the angle of the location’s latitude (in New Jersey, this is approximately 40°N).
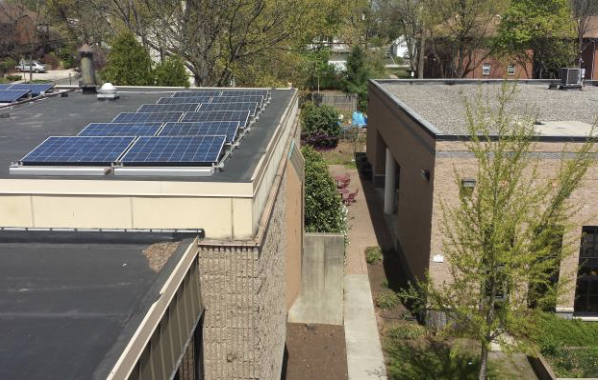
Figure 2 – Borough Hall, Highland Park, NJ. (Source: Rutgers Center for Green Building).
Geothermal
Geothermal heat pumps (GHPs), also known as ground source heat pumps or geo-exchange systems, take advantage of the Earth’s relatively constant temperature (45°F- 75°F),[4] cooling buildings in the summer and warming them in the winter. Some geothermal systems have wells drilled vertically deep underground, while others have horizontal loops a shorter distance below ground.[5] ENERGY STAR qualified geothermal heat pumps use about 45% less energy than a standard heat pump.[6] Underground piping carries warranties of 25–50 years, heat pumps often last 20 years or more.[7]
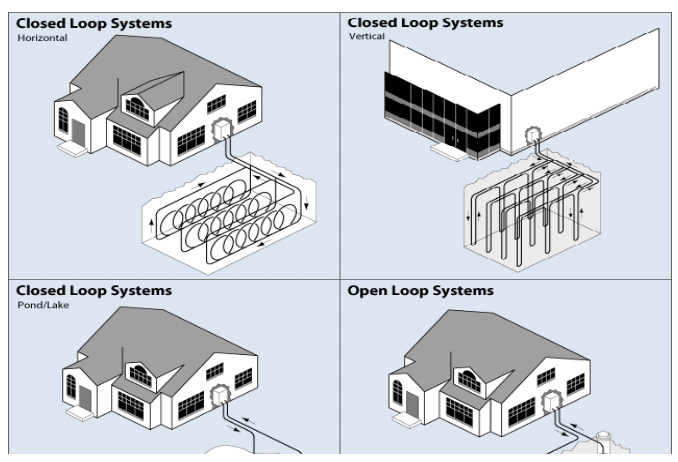
Geothermal Heat Pump Systems with Associated Equipment. (Source: Whole Building Design Guide: Geothermal Heat Pumps)
Wind Power
Wind systems provide renewable energy in areas with sufficient wind speed. Prime locations for harvesting wind energy in New Jersey are along the shore. [8]
The two main types of wind turbines include horizontal axis wind turbines (HAWT), the most common, and vertical axis wind turbines (VAWT).[9] Initially designed to be mounted on high poles in open or rural areas, small wind turbines (SWTs) include systems that are 100 kilowatts (kW) or less. Built-environment wind turbines (BEWT) refer to projects constructed on, in, or near buildings.[10] The highly turbulent and variable wind directions often found in the built environment make VAWTs a viable option as VAWTs do not need to orient into the wind to generate power.[11] VAWTs come in two different design types: the Savonius design, which generates power through drag, and the Darrieus, which generates power through an aerodynamic lift.[12]
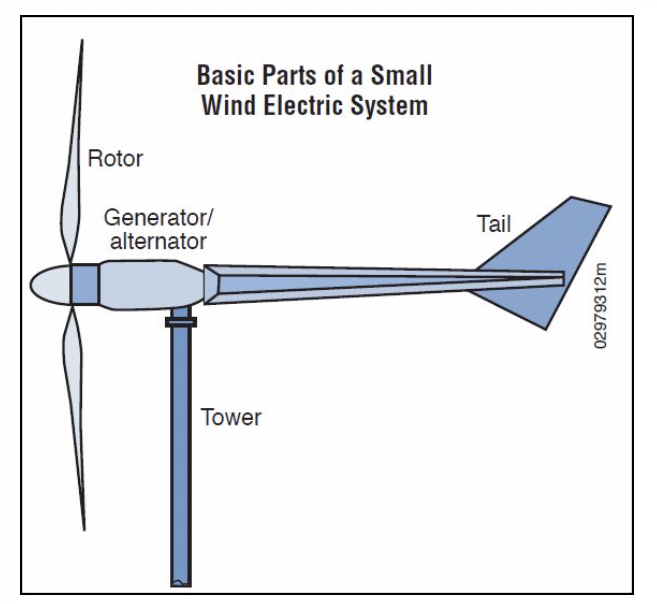
Figure 4. Small Wind Electric System (Source: US NREL).
Solar Thermal
Solar thermal, whether passive or active, utilizes solar power to heat water for domestic or process uses. Solar thermal water heating systems use tanks and solar collectors. Active water systems also use pumps and controls. Three common types of solar collectors include flat-plate collectors, integral collectors, and evacuated tube collectors. Flat-plate collectors consist of insulated and weatherproof boxes with a dark absorber plate under glass or plastic covers that heats water. Integral collectors use the same system as a flat-plate collector, but the water continues on to a traditional hot water tank, ensuring a consistent supply of hot water. Evacuated tube collectors consist of rows of transparent tubes that allow water to pass through and heat quickly.[13]
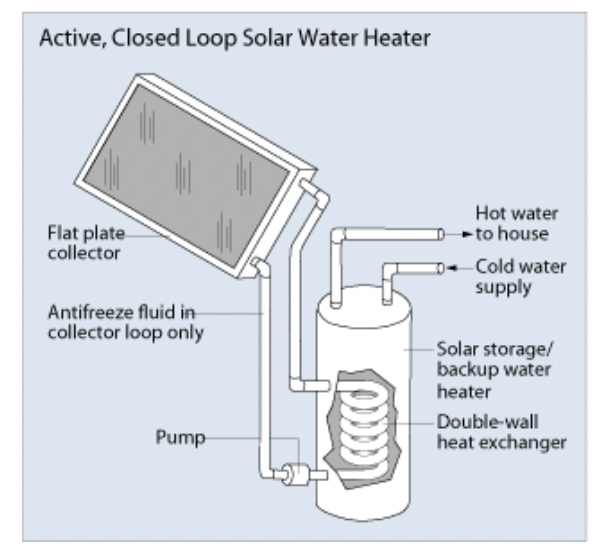
Figure 5 – Solar Water Heater (Source: US DOE | Energy Savers: Solar Water Heaters)
Biomass
Biomass energy systems utilize biomass, a renewable resource, to power facility heating, electric power generation, or combined heat and power (CHP) systems (see Combined Heat and Power (CHP)).[14] Biomass sources include organic material such as plant matter, wood, waste wood, residue from agriculture or forestry and organic matter from municipal or industrial waste. Fumes from landfills such as methane, a natural gas, provide biomass for electricity generation.[15]
In addition to purely biomass-powered electricity generation, consider biomass co-firing, applicable to commercial buildings that already have coal-fired boilers. Coal-fired boilers can handle a pre-mixed combination of coal and biomass. Alternatively, retrofit existing coal-fired boilers with a separate feed system for the biomass allowing biomass and coal to mix inside the boiler.[16]
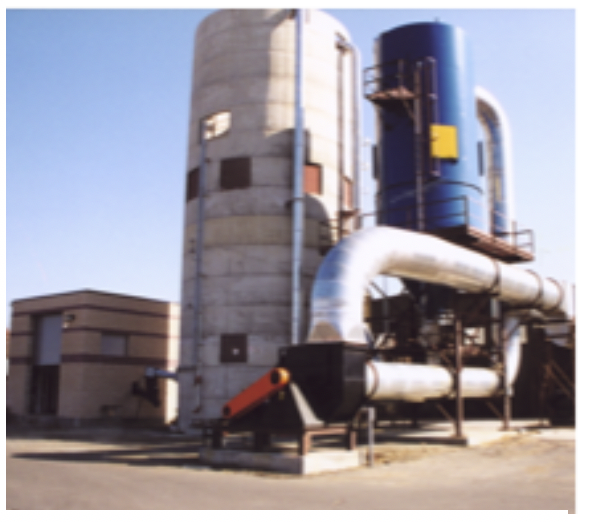
Figure 6 – Rex Lumber Biomass Facility, Englishtown, NJ. (Source: NJ Office of Clean Energy).
Examples
Geothermal: Atlantic County Utilities Authority (ACUA), Atlantic City/Egg Harbor Twp., NJ.
The ACUA’s clean energy initiatives include a geothermal heating and cooling system at its GeoBuilding. The system depends on heating well water through fifty closed-looped wells on a field adjacent to the building. The system extracts heat from the water in the winter and transfers heat to the ground in the summer.
PV: Solar Islanding Project, Highland Park, NJ.
This report analyzes the power demands and associated functions potentially sustained by three existing solar installations in Highland Park, NJ if converted into solar power islands. The report includes recommendations in language consistent with the National Incident Management System (NIMS) standard for adoption by other municipalities who may already have solar islands.
Vineland Solar One, Vineland, NJ.
Located on 14 acres at the Landis Sewage Authority (LSA), the Vineland Solar One generation facility includes 13,456 monocrystalline silicon panels that produce up to 5,500 MWh of energy annually. The NJ Office of Clean Energy Solar Renewable Energy Credit (SREC) Program and a Power Purchase Agreement between the Vineland Municipal Electric Utility (VMEU) and Conectiv Energy helped finance this public/private partnership.
Wind: Jersey Atlantic Wind Farm, Atlantic City, NJ.
The Jersey-Atlantic Wind Farm, a community-scale wind turbine installation, includes five, 380-foot turbines, producing a combined 7.5 megawatts of power. The wind turbines and on-site solar installation power the wastewater treatment plant, with surplus energy sent to the grid.
This study looks at several case studies of Built Environment Wind Turbines (BEWTs), documenting mixed-results in the BEWT industry. Main obstacles cited include, 1) insufficient and not well-defined project feasibility and planning processes, 2) high-cost projects related to additional expenses for installation and operations in the built environment, 3) a lack of certified manufacturers for ongoing service and parts, and 4) over-estimated project performance due to the complexity of conducting accurate resource and production assessments in the built environment. BEWT projects with the best outcomes had well developed and quantified project goals that included energy production as well as educational and sustainability marketing goals. These projects also conducted thorough feasibility investigations, used certified HAWTs with a proven track record, and were installed on tall buildings relative to surrounding obstacles.
Solar Thermal: Solar Water Heating in Multifamily Building, Greenfield, MA.
The Consortium for Advanced Residential Buildings (CARB), a US DOE Building America team, installed a solar domestic hot water (SDHW) system (372 ft2 evacuated tub, 330 gallons storage) as part a major renovation project that converted an old school building into 12 high-performance apartments.
Biomass: Biomass Energy Resource Center (BERC)
With funding from the US DOE, the Biomass Energy Resource Center (BERC) developed a series of 55 case studies illustrating the design and operational experiences of various community-scale facilities, including businesses, campuses, community buildings, schools, housing and government facilities, that employ biomass systems in the US, Canada, Europe, and Scandinavia.
The Rex Lumber Company, Englishtown, NJ.
The Rex Lumber Company, with a rebate for nearly 50% from the NJ Clean Energy Program, replaced a natural gas system with a wood-waste boiler biomass system to heat its kilns.
Benefits
Incorporating on-site renewable energy systems reduces greenhouse gas emissions, protects against the fluctuating costs of fossil fuels, and saves on purchasing energy from utility companies while providing additional ecological and user benefits as detailed below.
Biomass energy systems turn waste into resources, provide a renewable source of electricity and reduce the use of and dependency on fossil fuels, reducing greenhouse gas emissions and enhancing fuel supply security. Biomass powered CHP systems have the added benefit of generating both low-carbon electricity and low-carbon heat, further increasing energy efficiency and reducing carbon emissions by utilizing waste heat and using fuel more efficiently.[17] Biomass reduces waste management costs like tipping fees while promoting local business and farming. Replacing up to 20% of fossil fuel such as coal with bio-fuel also reduces nitrous oxides, carbon dioxide, and sulfur dioxide emissions. Capturing methane, a gas emitted by the decomposition of waste 28-36 times stronger than carbon dioxide, reduces emissions of a significant greenhouse gas.[18]
Geothermal pumps have a higher efficiency than air pumps and produce less noise pollution as most of the moving parts are underground. Depending on the cost and type of fuel, heating with geothermal pumps can cost 25-50% less than that of conventional heating.[19] Compared to air-source heat pumps, geothermal pumps last longer, require less maintenance and do not depend on outside air temperatures.[20]
The modularity of PV systems allows great flexibility in applying this technology to buildings. Solar PV can help manage peak demand and lower utility rates, while also providing shade and urban heat island reduction benefits.
Solar thermal systems reduce water consumption as well as the consumption of consumable, non-renewable, and often non-domestic energy sources such as natural gas.[21]
Wind power reduces dependence on carbon-intensive fuels such as coal.[22] Generating power from wind reduces greenhouse gas (GHG) emissions and promotes the renewable energy industry in New Jersey.
Costs
Geothermal – The capital cost of geothermal systems average $7, 000.00 per ton including the bore field.[23] Highly site-specific, well field costs can quickly add tens of thousands of dollars to overall system cost. Life-cycle costs can deliver a relatively quick payback beginning at 5 years, based on significant heating cost savings.[24] Annual energy savings can vary from 25% to 50%.[25] Also, maintenance and upkeep cost less than traditional types of heating and cooling equipment.
- Incremental cost (e.g., the cost of the green technology minus the cost of conventional technology) is around $3,957/ton.[26]
- Annual cost savings (e.g., annual kWh saved * $0.14/kWh [the price of electricity])
- According to US DOE Energy Savers, using GHPs reduce electricity consumption by 25-50%.
Scenario: Consider a 20,000 SF commercial building using 50,000[27] btu/SF over five years.
In one year: 50,000btu/SF ÷ 5 years = 10,000 btu
10,000 btu/SF * 20,000 SF = 200,000,000 btu
200,000,000 btu * (2.93×10-4 kWh/1 btu) = 58,614kWh consumed.
A reduction in electricity consumption of 50% means a savings of 29,307 kWh, or:
29,307 kWh * $0.14/kWh = $ 4,102.98
- Payback (Capital Costs or Incremental Costs / annual cost savings)
Incremental Cost = $3,957
Annual Cost Saving = $4,102.98
$3,957/$4,102.98 = 0.96
It will take less than one year to achieve a return on the investment in a geothermal heat pump.
Photovoltaic – PV systems have medium to high first costs, but like geothermal systems, have a quick payback, facilitated by various incentives and renewable energy credits. The New Jersey Office of Clean Energy Solar Renewable Energy Certificate (SREC) program provides renewable energy credits (RECs) during the first 15 years of the system’s life. Credits accumulate whenever a solar installation generates 1000 kWh of electricity. Prices for SREC credits sold through the SREC tracking system vary due to supply and demand.[28] System costs vary depending on type of panel used, roof type, and size of the system. In New Jersey, a commercial system typically cost between $3 and $5 a Watt, including installation. [29] Recent SREC pricing information can be found here: http://www.njcleanenergy.com/renewable-energy/project-activity-reports/srec-pricing/srec-pricing
Small Wind – Small wind installations cost about $800 to $1100 per kilowatt of generating capacity.[30] The price of a system varies depending on the size and details of the service agreement. Depending on the strength of the wind resource and the cost of the system, the typical paybacks range from 6-15 years.
Solar Thermal – The average price of solar thermal collector equipment varies depending on the type (low, medium, high temperature) and ranges from $1.94-$25.32 per square foot, averaging $7.01 per square foot.[31] The cost table below compares the average cost of solar thermal to conventional energy sources for 2017.
New Jersey | Electricity | Natural Gas | Solar thermal |
Average Cost per kilowatt-hour in commercial and industrial sectors: | 10.16-12.31¢[32] | 9.05¢[33] | 9.2-12¢[34] |
Biomass – The economics of biomass power generation depends on the availability of a predictable, local, sustainably-sourced, low-cost and long-term feedstock supply.[35] Differences in feedstock chemical composition impact the cost of the feedstock. For example, waste produced due to industrial processes can have a zero or even negative cost by avoiding waste disposal charges. Likewise, prices for dedicated energy crops can spike due to low productivity and high transportation costs. Lower cost options may include agricultural, and forestry residues collected and transported over short distances or available locally as a by-product.
The wide range of bioenergy-fired power generation technologies translates into a broad range of installed costs. For example, installation costs for biomass heating plants average between $500 to $1500 per kW-thermal of installed heating rate capacity.[36] Installation for small-scale biomass electric plants typically cost between $3,000 to $4,000 per kW.[37]
Fixed operations and maintenance (O&M) costs, such as labor, scheduled maintenance, routine component or equipment replacement, and insurance for bioenergy power plants typically range from 2-6% of total installed costs per year, while variable O&M costs average 0.005/KWh.[38]
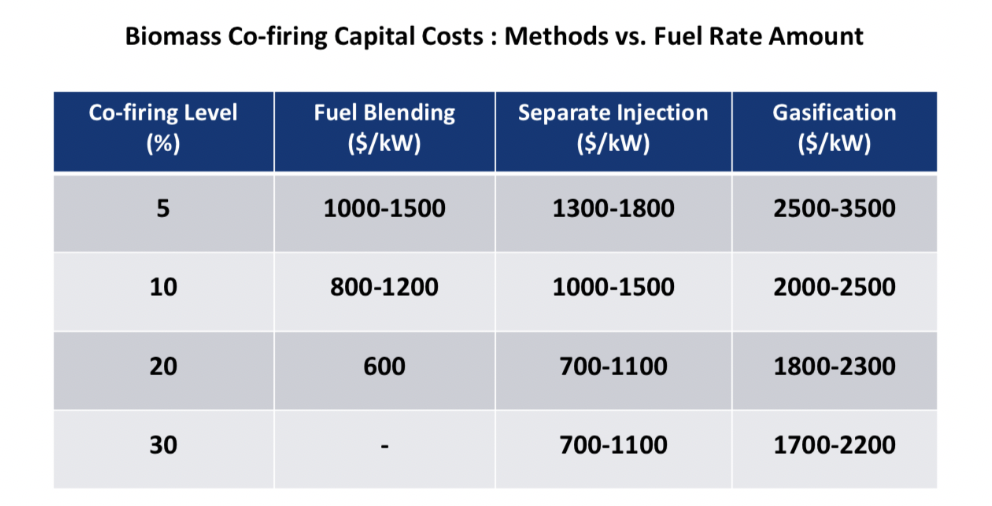
Source: NREL
Resiliency
On-site renewable energy systems promote resiliency in many ways. They tend to rely on site-based “fuel” and may island or operate independently from the power grid (see Solar Islanding and Microgrid Ready Solar PV, Energy Storage and Back-up Power Generation). For example, solar islanding and microgrid ready PV systems with battery storage combine the benefit of traditional PV systems, which avoid the use of fossil fuels, while also providing a resilient, local, and independent source of electric power during power emergencies and help to manage peak demand and lower utility costs throughout the year via demand response programs. By providing localized power generation, islandable PV systems increase energy efficiency by reducing transmission and distribution losses typical of centralized grid systems.[39] Unlike diesel and fossil-fueled generators, typically used only during emergencies, islandable PV systems with battery storage provide value throughout the year, do not require regular maintenance or refueling, and can provide emergency power for extended periods of time given available solar resources and adequate battery storage.[40] Some systems provide additional protection from flooding events, based on elevated installations, such as PV roof or pole-mounted systems.
For recommendations on how to incorporate solar islanding capabilities into existing facilities, see the Highland Park Solar Islanding Project.
Biomass offers several resiliency benefits including the ability to stockpile fuel for emergencies.[41] Plans to recover, segregate, and process biomass fuel locally makes downed biomass from fallen trees and vegetation caused by storms and natural disasters a cost-saving resource rather than a post-disaster clean-up expense.[42] Making use of downed biomass also frees up time and money for other recovery efforts, while at the same time providing short to long-term backup power for critical infrastructure and emergency services.
Biomass energy systems with off-grid or islanding capabilities and thermal energy storage provide enhanced energy independence, security, and reliability in the event of natural disasters and security threats while also helping to manage peak demand and lower utility costs throughout the year via demand response programs (see Thermal Energy Storage, Battery Storage and Back-up Power Generation, Demand Response and Peak Load Management).[43] Biomass-fired CHP systems promote the renewable energy industry in New Jersey by utilizing renewable sources of fuel, providing backup power and balancing the supply and demand of electricity from variable renewables such as wind and solar.[44] A form of distributed energy generation, biomass-fired CHP systems support the development and integration of microgrids and the broader smart grid, which aims to diversify and strengthen the electric grid through better energy management and the integration of cleaner energy sources and energy storage.
[1] US Dept of Energy’s (DOE) Federal Energy Management Program (FEMP). Guide to Integrating Renewable Energy in Federal Construction. https://www.wbdg.org/FFC/DOE/DOECRIT/re_construction_guide.pdf (accessed May 8, 2018).
[2] Eiffert Ph. D, Patrina and Gregory J. Kiss. NREL. Building-Integrated Photovoltaic Designs for Commercial and Institutional Structures: A Sourcebook for Architects.” www.nrel.gov/docs/fy00osti/25272.pdf (accessed April 5, 2018).
[3] NJ Clean Energy Program. Renewable Energy: Programs: SREC Registration Program. http://www.njcleanenergy.com/renewable-energy/programs/solar-renewable-energy-certificates-srec/new-jersey-solar-renewable-energy (accessed April 12, 2018).
[4] US DOE “Geothermal Heat Pumps.” https://www.energy.gov/eere/geothermal/geothermal-heat-pumps (accessed April 4, 2018).
[5] US DOE. “Guide to Geothermal Heat Pumps” https://www.energy.gov/sites/prod/files/guide_to_geothermal_heat_pumps.pdf (accessed April 4, 2018).
[6] ENERGY STAR. “Heat Pumps, Geothermal for Consumers.” http://www.energystar.gov/index.cfm?fuseaction=find_a_product.showProductGroup&pgw_code=HP (accessed April 4, 2018).
[7] US DOE. “Benefits of Geothermal Heat Pump Systems.” https://www.energy.gov/energysaver/choosing-and-installing-geothermal-heat-pumps (accessed April 4, 2018).
[8]American Wind Energy Association. “Wind 101: Wind Energy Basics.” https://www.awea.org/wind-power-101 (accessed April 11, 2018).
[9] WBDG. Wind Technology. https://www.wbdg.org/resources/wind-technology (accessed April 19, 2018).
[10] Jason Fields, Frank Oteri, Robert Press, and Ian Baring-Gould (2016) National Renewable Energy Laboratory. Deployment of Wind Turbines in the Built Environment: Risks, Lessons, and Recommended Practices https://www.nrel.gov/docs/fy16osti/65622.pdf (accessed April 20, 2018).
[11] J. Smith, T. Forsyth, K. Sinclair, and F. Oteri. (2015) NREL. Built-Environment Wind Turbine Roadmap. https://www.nrel.gov/docs/fy13osti/50499.pdf (accessed April 20, 2018).
[12] WBDG. Wind Technology. https://www.wbdg.org/resources/wind-technology (accessed April 19, 2018).
[13] US DOE. Solar Water Heaters. https://www.energy.gov/energysaver/water-heating/solar-water-heaters (accessed April 12, 2018).
[14] Whole Building Design Guide (WBDG): Biomass to Heat. https://www.wbdg.org/resources/biomass-heat (accessed April 30, 2018).
[15] NJ Clean Energy Program. “Biopower.” http://www.njcleanenergy.com/renewable-energy/technologies/biopower/biopower (accessed April 12, 2018).
[16] US EIA “Biomass for Electricity Generation.” 2002. http://www2.et.byu.edu/~tom/classes/733/Biomass/Biomass%20for%20electricity%20generation.pdf (accessed April 12, 2018).
[17] International Energy Agency (IEA). 2011. Co-generation and Renewables – Solutions for a low-carbon energy future. https://www.iea.org/publications/freepublications/publication/CoGeneration_RenewablesSolutionsforaLowCarbonEnergyFuture.pdf (accessed Oct 9, 2018).
[18] US EPA. https://www.epa.gov/lmop/basic-information-about-landfill-gas (accessed April 30, 2018).
[19] US DOE. Energy Savers: Benefits of Geothermal Heat Pumps. https://www.energy.gov/energysaver/choosing-and-installing-geothermal-heat-pumps (accessed April 20, 2018).
[20] US DOE | EERE. “Geothermal Heat Pumps.” https://www.energy.gov/energysaver/choosing-and-installing-geothermal-heat-pumps (accessed April 4, 2018).
[21] Denholm, P. 2007. “The Technical Potential of Solar Water Heating to Reduce Fossil Fuel Use and Greenhouse Gas Emissions in the United States.” National Renewable Energy Laboratory, Golden CO. https://www.nrel.gov/docs/fy07osti/41157.pdf (accessed April 23, 2018).
[22] McCollum, David, and Christopher Yang. 2009. “Achieving deep reductions in US transport greenhouse gas emissions: Scenario analysis and policy implications.” Energy Policy 37: 5580-5596.
[23] Rutgers University Facilities Department – Based on estimates received for new buildings.
[24] US DOE. “Geothermal Heat Pumps.” https://www.energy.gov/energysaver/heat-and-cool/heat-pump-systems/geothermal-heat-pumps (accessed April 18, 2018).
[25] US DOE. Choosing and Installing a Geothermal Heat Pump System. https://www.energy.gov/energysaver/choosing-and-installing-geothermal-heat-pumps (accessed April 12, 2018).
[26] NEEP. “Mid-Atlantic Technical Reference Manual Version 7.” http://neep.org/sites/default/files/resources/Mid_Atlantic_TRM_V7_FINAL.pdf (accessed April 18, 2018).
[27] US DOE | EERE. Planning, Budget & Analysis: Commercial Buildings Total Energy Consumption. April 2008. https://www.energy.gov/eere/analysis/energy-intensity-indicators Assume an average of 50k btu for all sectors. (accessed April 18, 2018).
[28] NJ OCE. SREC Registration Program. http://www.njcleanenergy.com/renewable-energy/programs/solar-renewable-energy-certificates-srec/new-jersey-solar-renewable-energy (accessed April 19, 2018).
[29] NJ OCE. NJCEP Solar Project data
[30] US DOE. 2016 Wind Technologies Market Report. https://www.energy.gov/sites/prod/files/2017/10/f37/2016_Wind_Technologies_Market_Report_101317.pdf (accessed April 19, 2018).
[31] US DOE | EIA. Form EIA-63A, “Annual Solar Thermal Collector Manufacturers Survey.” From table 2.12 at https://www.eia.gov/renewable/annual/solar_thermal/ (accessed April 23, 2018).
[32] US DOE | EIA. “Electricity Data Browser.” https://www.eia.gov/electricity/data/browser/#/topic/7?agg=0,1&geo=g004&endsec=u&linechart=ELEC.PRICE.US-ALL.A&columnchart=ELEC.PRICE.US-ALL.A&map=ELEC.PRICE.US-ALL.A&freq=A&ctype=linechart<ype=pin&rtype=s&maptype=0&rse=0&pin (accessed April 23, 2018).
[33] US DOE | EIA. “Natural Gas Prices.” 2017 Available for Commercial only https://www.eia.gov/dnav/ng/ng_pri_sum_dcu_SNJ_a.htm (accessed April 23, 2018).
[34] NREL. The U.S. Solar Photovoltaic System Cost Benchmark: Q1 2017. https://www.nrel.gov/news/press/2017/nrel-report-utility-scale-solar-pv-system-cost-fell-last-year.html (accessed April 23, 2018).
[35] International Renewable Energy Agency (IREA). Renewable Power Generation Costs in 2017 http://www.irena.org/publications/2018/Jan/Renewable-power-generation-costs-in-2017 (accessed April 27, 2018).
[36] WBDG. Biomass from Heating. https://www.wbdg.org/resources/biomass-heat (accessed April 27, 2018).
[37] WBDG. Biomass from Electricity. https://www.wbdg.org/resources/biomass-electricity-generation (accessed April 27, 2018).
[38] IREA. Renewable Power Generation Costs in 2017 http://www.irena.org/publications/2018/Jan/Renewable-power-generation-costs-in-2017 (accessed April 27, 2018).
[39] E. Hotchkiss, I. Metzger, J. Salasovich, and P. Schwabe. 2013 Alternative Energy Generation Opportunities in Critical Infrastructure New Jersey. Produced under the direction of the U.S. Federal Emergency Management Agency by the National Renewable Energy Laboratory (NREL) https://www.nrel.gov/docs/fy14osti/60631.pdf (accessed Oct 3, 2018).
[40] NREL. 2018. “Valuing the Resilience Provided by Solar and Battery Energy Storage Systems.” https://www.nrel.gov/docs/fy18osti/70679.pdf (accessed Oct 3, 2018).
[41] WBDG. Biomass from Heating. https://www.wbdg.org/resources/biomass-heat (accessed April 27, 2018).
[42] Ibid.
[43] Woody Biomass Utilization Working Group (WBUG). Benefits of Biomass. https://www.forestsandrangelands.gov/Woody_Biomass/index.shtml (accessed April 27, 2018).
[44] International Energy Agency (IEA). 2011. Co-generation and Renewables – Solutions for a low-carbon energy future. https://www.iea.org/publications/freepublications/publication/CoGeneration_RenewablesSolutionsforaLowCarbonEnergyFuture.pdf (accessed Oct 9, 2018).
Resources
- Database of State Incentives for Renewables & Efficiency (DSIRE)
- ENERGY STAR Geothermal Heat Pumps
- International Renewable Energy Agency (IREA)
NJ Clean Energy
US DOE
- Bioenergy Technologies Program
- Geothermal Technologies Office
- Solar Energy Technologies Office
- Wind Energy Technologies Office
US DOE’s Federal Energy Management Program (FEMA)
Whole Building Design Guide (WBDG)
- Biomass for Heat
- Building Integrated Photovoltaics (BIPV)
- Geothermal Heat Pumps
- Solar Water Heating
- Wind Technology
Tools