Combined Heat and Power (CHP)
Existing CommercialWhat is Combined Heat and Power (CHP)?
Combined Heat and Power (CHP), also known as cogeneration, is a type of distributed energy resource that produces both electricity and thermal energy onsite, using one primary fuel.[1] CHP recovers heat usually wasted in conventional power generation as thermal energy for a building’s space heating and cooling, process heating and cooling, dehumidification, or the production of more electricity generation.[2] CHP system can use fossil or renewable fuels and typically utilize the following prime mover technologies to combust the fuel including reciprocating engines, gas turbines, microturbines, fuel cells, and boiler or steam turbines.
For example, (see Figure 1) a prime mover, such as a reciprocating engine or gas turbine uses a fuel such as natural gas to power a generator that creates electricity and emits heat. Generated electricity supplies electrical loads in the building, feeds back to the grid, or utilizes energy storage for later use (see Energy Storage and Backup Power Generation). A heat recovery unit captures the heat and processes it for use onsite, typically for building heating applications but also for absorption cooling, an application that utilizes heat energy to cool buildings.[3] CHP systems can utilize thermal energy storage to save excess heat energy for later use when it is needed, such as during a power outage, to increase energy efficiency, and to store low priced energy and use that energy during peak demand when prices climb (see Thermal Energy Storage).[4]
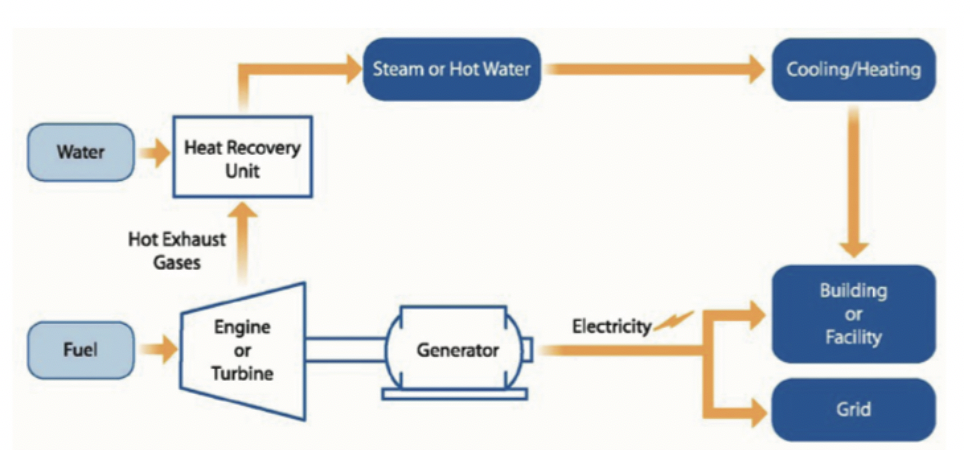
Figure 1. Reciprocating engine or gas turbine with heat recovery. (Source: US DOE CHP)
Microturbines use gaseous or liquid fuels, most commonly natural gas, to produce electricity and recover exhaust heat for direct use or use in a heat recovery boiler.[5] Steam turbines do not consume fuel directly but use steam generated by natural gas, oil, coal or biomass driven boilers or heat recovery steam generators, to produce power and thermal energy (see On-Site Renewable Energy Systems).[6]
Fuel cells used for CHP applications utilize an electrochemical process to reform natural gas or biogas into hydrogen and then react hydrogen to generate electricity.[7]
How to Integrate CHP
The practicality of integrating CHP into a project increases when considered early in the planning stages, including early engagement with the local utility to identify permitting issues and available incentives.
Contact the NJ Office of Clean Energy to learn about current CHP programs, tools, and available funding. For example, the NJ Office of Clean Energy Combined Heat and Power (CHP) Program currently offers incentives for the following CHP technologies:[8]
- Powered by non-renewable (i.e., natural gas) or renewable fuel source (i.e., biomass).
- Gas internal combustion engine.
- Gas combustion turbine.
- Microturbine
- Fuel cells with heat recovery.
- Waste heat to power.
The use of Class 1 renewable fuel sources and installation of CHP with black-start and islanding capabilities at designated critical facilities may qualify projects for additional incentives.
Example
Westin – Starwood Hotel, Jersey City, NJ
The Westin Jersey City Newport qualified for the NJ Clean Energy Combined Heat & Power Program by installing three Tecogen® Inc. 100-kW natural gas engine CHP units. The co-generation units are expected to produce 1,985 MWh of electricity and 132,962 therms of energy per year. The Westin Jersey City Newport qualified for $600,000 in NJCEP incentives, covering more than 27 percent of the $2,158,229 project cost, which includes ten years of maintenance service by the manufacturer. The co-generation units could potentially save the hotel, on average, 367,209 kWh of electricity and 91,198 therms of natural gas per year, resulting in annual savings ranging from $123,007 to $212,000. With the NJCEP incentives, the project expects to pay for itself within a decade when factoring in federal tax credits.
Benefits
Inherently inefficient, conventional electricity generation converts only about a third of a fuel’s potential energy into usable energy.[9] It is reasonable to expect CHP applications to operate at 65-75% efficiency, a large improvement over the national average of ~50% for these services when separately provided.[10] Since overgeneration of heat and electricity occurs frequently, CHP systems that utilize both thermal and electrical storage further increase energy efficiency by capturing wasted energy.[11] The significant increase in energy efficiency with CHP means lower fuel consumption and reduced greenhouse gas emissions for the same level of output when compared to the separate generation of heat and power. Biomass powered CHP systems have the added benefit of generating both low-carbon electricity and low-carbon heat, further increasing energy efficiency and reducing carbon emissions by utilizing waste heat and using fuel more efficiently (see On-Site Renewable Energy Systems).[12] Other benefits include CHP’s proven track record and the versatility of CHP to work with existing and planned technologies for various industrial and commercial applications. CHP also localizes energy generation, avoiding congestion issues and improving grid transmission and distribution losses associated with centralized power stations, reducing the risk of electrical grid disruptions and enhancing energy reliability. As a distributed energy generator, CHP provides a reliable and independent source of electric power during power emergencies and grid outages and can help to manage peak demand and lower utility costs throughout the year via demand response programs (see Demand Response and Peak Load Management).
Costs
CHP makes the most economic sense when electricity prices are high, and natural gas prices are low. While the initial cost of CHP systems often exceed that of conventional heating or cooling systems, reduced energy and operating costs associated with cogeneration can often justify the investment.
Resiliency
CHP can improve the resiliency of critical infrastructure, by acting as a self-sufficient microgrid keeping essential facilities and systems running without any interruption in electric or thermal service in the absence of utility sourced power due to storms or other events.[13]
For example, South Oaks Hospital in Amityville, New York remained open and operational during Superstorm Sandy while over 8.5 million Long Island Power Authority (LIPA) customers had no power for a week.[14] South Oaks Hospital had five 250 kW units, which kept lights on and refrigeration units operational for 15 days, even allowing the hospital to admit new patients.
A form of distributed energy generation, CHP systems support the development and integration of microgrids with the broader smart grid by providing a consistent and reliable source of backup power that helps to balance the supply and demand of electricity from other distributed energy generators, including variable renewables such as wind and solar.[15] Combined with energy storage capabilities, CHP systems hold the potential to help diversify and strengthen the electric grid through better energy management and the integration of distributed energy resources and energy storage (see Thermal Energy Storage, Energy Storage and Backup Power Generation).
[1] US EPA and US DOE. Guide to Using Combined Heat and Power for Enhancing Reliability and Resiliency in Buildings. September 2013. https://www.energy.gov/sites/prod/files/2013/11/f4/chp_for_reliability_guidance.pdf (accessed April 18, 2018).
[2] E. Hotchkiss, I. Metzger, J. Salasovich, and P. Schwabe. 2013. Alternative Energy Generation Opportunities in Critical Infrastructure New Jersey. Produced under the direction of the U.S. Federal Emergency Management Agency by the National Renewable Energy Laboratory (NREL) https://www.nrel.gov/docs/fy14osti/60631.pdf (accessed Oct 3, 2018).
[3] International Energy Agency (IEA). 2011. Co-generation and Renewables – Solutions for a low-carbon energy future. https://www.iea.org/publications/freepublications/publication/CoGeneration_RenewablesSolutionsforaLowCarbonEnergyFuture.pdf (accessed Oct 9, 2018).
[4] Energy Storage Association. 2018. Distribute Grid-Connected PV Integration
http://energystorage.org/energy-storage/technology-applications/distributed-grid-connected-pv-integration (accessed Oct 18, 2018).
[5] US DOE. 2017. Combined Heat and Power Fact Sheets: Microturbines https://www.energy.gov/sites/prod/files/2016/09/f33/CHP-Microturbines_0.pdf (accessed Oct 4, 2018).
[6] US DOE. 2017. Combined Heat and Power Fact Sheets: Steam Turbines. https://www.energy.gov/sites/prod/files/2016/09/f33/CHP-Steam%20Turbine.pdf (accessed Oct 4, 2018).
[7] US DOE. 2017. Combined Heat and Power Fact Sheets: Fuel Cells https://www.energy.gov/sites/prod/files/2016/09/f33/CHP-Fuel%20Cell.pdf (accessed Oct 4, 2018).
[8] NJ Office of Clean Energy. 2018. Combined Heat and Power (CHP) Program. http://www.njcleanenergy.com/commercial-industrial/programs/combined-heat-power/combined-heat-power (accessed Oct 5, 2018).
[9] Electropaedia. http://www.mpoweruk.com/fossil_fuels.htm (accessed April 12, 2018).
[10] DOE. Combined Heat and Power Basics. https://www.energy.gov/eere/amo/combined-heat-and-power-basics (accessed April 12, 2018).
[11] International Energy Agency (IEA). 2011. Co-generation and Renewables – Solutions for a low-carbon energy future.https://www.iea.org/publications/freepublications/publication/CoGeneration_RenewablesSolutionsforaLowCarbonEnergyFuture.pdf (accessed Oct 9, 2018).
[12] International Energy Agency (IEA). 2011. Co-generation and Renewables – Solutions for a low-carbon energy future. https://www.iea.org/publications/freepublications/publication/CoGeneration_RenewablesSolutionsforaLowCarbonEnergyFuture.pdf (accessed Oct 9, 2018).
[13] ICF International. 2013. “Combined Heat and Power: Enabling Resilient Energy Infrastructure for Critical Facilities.” Prepared for Oak Ridge National Laboratory. March 2013. Page 2. https://www.energy.gov/sites/prod/files/2013/11/f4/chp_critical_facilities.pdf (accessed April 12, 2018).
[14] ACEEE. New and Improved Methods for Evaluating CHP Cost-Effectiveness. https://aceee.org/files/proceedings/2016/data/papers/4_837.pdf (accessed April 12, 2018).
[15] International Energy Agency (IEA). 2011. Co-generation and Renewables – Solutions for a low-carbon energy future. https://www.iea.org/publications/freepublications/publication/CoGeneration_RenewablesSolutionsforaLowCarbonEnergyFuture.pdf (accessed Oct 9, 2018).