Life Cycle Cost Analysis (LCCA)
New CommercialWhat is Life Cycle Cost Analysis (LCCA)?
Life Cycle Cost Analysis (LCCA) is a form of economic evaluation that compares the costs and savings of alternative building designs and systems over the life cycle of a building. Green building features generally have higher initial costs compared to conventional features but offer operational savings over time through decreased resource use (e.g., energy, water), maintenance, and disposal fees.[1] LCCA takes into account the overall costs of purchasing, operating, maintaining, and disposing of a building or building system, which the initial purchase price does not reflect. Comparing the life cycle costs of various options helps determine the most cost-effective choice for a comparable building design or system in terms of function and performance by translating this data into dollar amounts and providing information about how long it takes an investment to pay for itself (e.g., internal rate of return, savings-to-investment ratio, payback period).
While LCCA’s take into account the life-cycle costs of building designs and systems, a Life-Cycle Assessment (LCA) evaluates the environmental impacts of products, services, and systems (see Life-Cycle Assessment (LCA)).[2] Together, LCCA’s and LCA’s provide the essential components of a comprehensive cost-benefit analysis.
How to Perform a Life Cycle Cost Analysis (LCCA)
Perform the LCCA early in the project and as part of an integrated design process to allow sufficient time and resources to refine the design and ensure the highest quality product for the lowest cost (see Integrated Design Process). Depending on project team expertise, hire a consulting service or conduct the LCCA in-house. Project teams should refer to the ASTM standard life-cycle cost method, which covers the costs of initial investment, replacement, operation, maintenance and repair, and disposal.[3]
The first task of an LCCA involves determining the costs and benefits of alternative project options. Next, project teams can conduct several sensitivity analyses that include variations in the discount rate, study period or building lifespan, and energy escalation rates.
The discount rate is a measure that decreases the value of dollars spent or received in the future, so future costs and returns are worth progressively less in terms of today’s dollars. The interest rate used for discounting is a rate that reflects an investor’s opportunity cost of money over time. The LCCA method converts cash flows to present values by discounting them to a common point in time, usually the base date.
The US Department of Energy (US DOE) suggests a real discount rate of 3.0%, based on a 12-month average of long-term Treasury bond rates.[4]
Study period or building lifespan refers to the length of time the LCC analysis covers. The study period for non-residential buildings typically falls between 20 and 40 years and may reflect a shorter period than the actual intended life of the facility.
Energy escalation rates refer to projected average annual energy price increases obtained either from the supplier or from energy price escalation rates published annually on April 1 by US DOE in Energy Price Indices and Discount Factors for Life-Cycle Cost Analysis, Annual Supplement to NIST Handbook 135.[5]
There are several online tools for performing an LCCA and links to more information in the Resources section below.
Example
New Jersey Meadowlands Commission: Observatory and Classroom Building LCCA, Lyndhurst, NJ.
The Rutgers Center for Green Building conducted an LCCA of the Meadowlands Environmental Center, a LEED Platinum building, modeling the building’s metabolic flows, including heating, lighting, ventilation, water, and power consumption, and waste production. The result was a quantification of any added capital investments encountered during construction and commissioning of the LEED-compliant structure, as well as a use-phase evaluation of the economic impacts of adherence to a green building standard.
Besides the Photovoltaic System (PV), the study results showed that daylight sensors provided the most cost-effective green feature, providing savings under all of the modeled situations as well as the most significant savings compared to other green features. The HVAC system projected savings consistently, although of lower value than the daylight sensors. Insulation provided savings under a majority of circumstances but showed a low return value in all cases. The study noted that the value of SREC’s directly impacts the cost-effectiveness of the photovoltaic (PV) system.
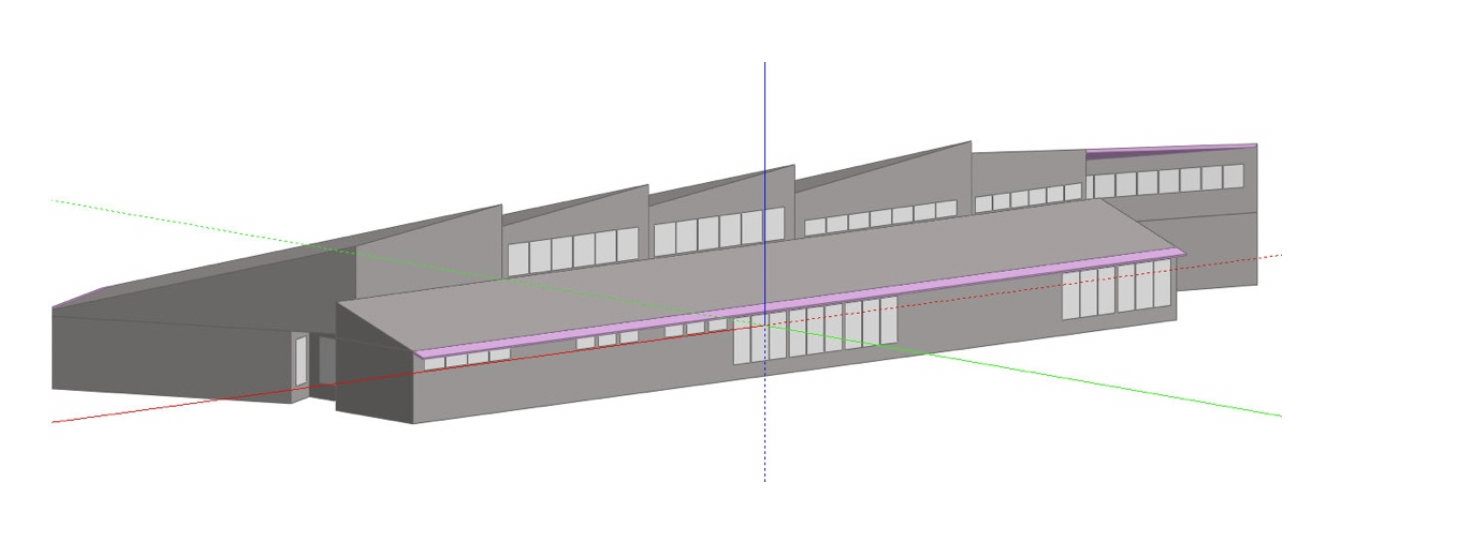
Figure 1 – Three-dimensional model of the New Jersey Meadowlands Commission Center for Environmental & Science Education (Source: Rutgers Center for Green Building).
Benefits
LCCA’s can offer environmental and monetary benefits for new construction projects by revealing the most environmentally-friendly and cost-effective solutions for the long run that purchase price alone cannot quantify. Potential benefits include reduced energy, water, and fuel consumption and cost savings on utility bills, maintenance, replacement, and end-of-life disposal costs. While an LCCA cannot remove all uncertainty surrounding a building project, the results equip the design team with the means to identify the optimal balance between green design and economic efficiency.
Costs
The costs for performing an LCCA depend on the scope and complexity of the building project and on whether in-house expertise or an independent consulting service completes the analysis. Several free online tools and resources are available to help owners and developers perform an initial LCC analysis (see Resources). Costs for performing an LCCA typically include labor costs for data collection, energy modeling (if necessary), LCCA calculations, and the interpretation and reporting of results.
Resiliency
An LCCA can identify and evaluate the costs and benefits of green building strategies that enhance building resiliency. While LCCAs and energy modeling typically use historical weather data, specific, regional, and forward-looking climate data can be used in energy models to inform resilient building design.[6] For example, in the NJMC study mentioned above, the team ran an energy model scenario according to low-emissions mid-century predictions of climate change developed by the Northeast Climate Impacts Assessment (NECIA).[7]
LCCA’s can take into account resiliency objectives. These may include specific design inputs to address increasing heat, more intense precipitation, or rising sea levels, including cooling and shading buildings, improving the efficiency of building envelopes, utilizing green roofs and landscape elements, planning for increased failure or reduced efficiency of electrical or mechanical systems, and providing passive solar cooling and ventilation features.[8] LCCA’s focused on resilient design can also consider the avoided costs or resiliency benefits of avoided power outages, such as reduced business closures, health, and safety hazards, food spoilage, and inconvenience from schedule disruptions.[9]
[1] Whole Building Design Guide (WBDG). 2016. Building Life-Cycle Cost (BLLC). http://www.wbdg.org/additional-resources/tools/building-life-cycle-cost-blcc (accessed January 30, 2019).
[2] National Renewable Energy Laboratory (NREL). Life Cycle Assessments – U.S. Life Cycle Inventory Database. https://www.nrel.gov/lci/assessments.html (accessed January 30, 2019).
[3] ASTM E917-17 Standard Practice for Measuring Life-Cycle Costs of Buildings and Building Systems. https://www.astm.org/Standards/E917.htm (accessed January 27, 2019).
[4] This rate for life-cycle cost analyses is valid through March 31, 2019, according to US DOE. Current Discount Rates (accessed January 27, 2019).
[5] The National Institute of Standards and Technology (NIST). 2018. Energy Price Indices and Discount Factors for Life-Cycle Cost Analysis – 2018: Annual Supplement to NIST Handbook 135. https://www.energy.gov/eere/femp/building-life-cycle-cost-programs (accessed January 27, 2019).
[6] NYC Mayor’s Office of Recovery and Resiliency. April 2018. Climate Resiliency Design Guidelines. Version 2.0. Page 4. http://www1.nyc.gov/assets/orr/pdf/NYC_Climate_Resiliency_Design_Guidelines_v2-0.pdf (accessed June 11, 2018).
[7] Krogmann, U., Minderman, N., Senick, J. and Andrews, C.J. “Life-Cycle Assessment of the New Jersey Meadowlands Commission Center for Environmental and Scientific Education Building.” 2008. Prepared by Rutgers Center for Green Building for New Jersey Meadowland Commission. At http://rcgb.rutgers.edu/wp-content/uploads/2018/06/LCC-Final-Report-5-21-08.pdf(accessed June 19, 2018).
[8] NYC Mayor’s Office of Recovery and Resiliency. April 2018. Climate Resiliency Design Guidelines. Version 2.0. Page 9-19. http://www1.nyc.gov/assets/orr/pdf/NYC_Climate_Resiliency_Design_Guidelines_v2-0.pdf (accessed June 11, 2018).
[9] DOE. “Smart Grid Investments Improve Grid Reliability, Resilience, and Storm Responses.” https://www.smartgrid.gov/files/B2-Master-File-with-edits_120114.pdf(accessed May 1, 2018).
Related Strategies
Resources
- ASTM E917-17 Standard Practice for Measuring Life-Cycle Costs of Buildings and Building Systems
- Building for Economic and Environmental Software (BEES) – a free online tool developed by NIST for selecting cost-effective and environmentally preferable building products.
- General Service Administration (GSA) Life Cycle Costing
- US DOE Building Life Cycle Cost Programs and Tools
- R. S. Means Company – Construction Cost Databases
- The Sustainable Facilities Tool
- Whole Building Design Guide (WBDG): Design for Maintainability
- Whole Building Design Guide (WBDG): Life-Cycle Cost Analysis (LCCA)